Surface Plasmon Resonance
Surface Plasmon Resonance (SPR) is a physical process that can occur when plane-polarized light hits a thin metal film under total internal reflection conditions (1).
Total internal reflection
When a light beam hits a half circular prism, the light is bent towards the plane of interface, when it is passing from a denser medium to a less dense one. Changing the incidence angle (Θ) changes the out-coming light until it reaches a critical angle. At this point, all the incoming light reflects within the circular prism. This is called total internal reflection (TIR).
Although no light is coming out of the prism in TIR, the electrical field of the photons extends about a quarter of a wavelength beyond the reflecting surface.
Surface plasmons
Now the prism is coated with a thin film of a noble metal on the reflection site. In most cases, gold is used because it gives a SPR signal at convenient combinations of reflectance angle and wavelength. In addition, gold is chemically inert to solutions and solutes typically used in biochemical contexts (1). When the electrical field energy of the photon is just right, it can interact with the free electron constellations in the gold surface. These are the outer shell and conduction-band electrons. The incident light photons are absorbed and the energy is transferred to the electrons, which convert into surface plasmons.
Photon and electron behaviour can only be described when they have both wave and particle properties. In accordance with the quantum theory, a plasmon is the particle name of the electron density wave. Therefore, when in a TIR situation the quantum energy of the photons is right, the photons convert to plasmons leaving a 'gap' in the reflected light intensity.
Momentum resonance
Like all conversions, the photon to plasmon transformation must conserve both momentum and energy in the process. Plasmons have a characteristic momentum defined by factors that include the nature of the conducting film and the properties of the medium on either side of the film. Resonance occurs when the momentum of incoming light is equal to the momentum of the plasmons (momentum resonance).
The momentum of the photons and plasmons can be described by a vector function with both magnitude and direction. The relative magnitude of the components changes when the angle or wavelength of the incident light changes. However, as plasmons are confined to the plane of the gold film, for SPR it is only the vector component parallel to the surface that matters. Thus, the energy and the angle of incident light must match to create surface plasmon resonance.
Evanescent wave
In TIR, the reflected photons create an electric field on the opposite site of the interface. The plasmons create a comparable field that extends into the medium on either side of the film. This field is called the evanescent wave because the amplitude of the wave decreases exponentially with increasing distance from the interface surface, decaying over a distance of about one light wavelength (2). The depth of the evanescent wave which is useful for measurements is within ~300 nm of the sensor surface (3). The wavelength of the evanescent field wave is the same as that of the incident light. The energy of the evanescent wave is dissipated by heat. Equations that describe how electric fields travel through a medium include a term for the properties of the medium. For light, this term is the refractive index. The light is seen as refracted because the photons have a different velocity in different media.
In the same way, the velocity (and therefore the momentum) of the plasmons is changed when the composition of the medium changes. Because of the change in momentum, the angle of incident light at which the resonance occurs changes.
Light wave coupling
The coupling of the light wave to the surface plasmons can be monitored in several ways. The most commonly used set-up is the angular SPR, also known as resonant angle SPR (4). The resonant angle can be measured very precisely (5) and is for instance used by BIACORE. On the other hand, at a fixed angle of incident light, the wavelength can be varied until resonance occurs (6). This method is known as resonant wavelength SPR or spectral SPR and is not widely used (4). In addition, the phase shift of the light can be detected when the coupling between light and plasmons occur. This method uses a reference beam and has a high sensitivity but requires a more complex setup than other methods. A forth method of SPR monitoring is the measurement of the intensity of the reflected light by SPR imaging (7). This set-up makes it possible to simultaneously follow large numbers of SPR spots, and thereby increasing sample through put. Companies like IBIS TECHNOLOGIES and GWC TECHNOLOGIES market instruments in this area.
SPR dependencies
The surface plasmon resonance angle mainly depends on the properties of the metal film, the wavelength of the incident light and the refractive index of the media on either side of the metal film (1). Because the refractive index is sensitive to temperature, it is important to perform the measurements at defined temperatures. In some cases, this dependency can be exploited (8). The refractive index is not dependent on the density of the media (9).
The metal must have conduction band electrons capable of resonating with the incoming light at a suitable wavelength (10). Metals that satisfy this condition are silver, gold, copper, aluminium, sodium and indium. In addition, the metal on the sensor surface must be free of oxides, sulphides and it must not react with other molecules on exposure to the atmosphere or liquid (10). Of the metals, indium is too expensive, sodium too reactive, copper and aluminium too broad in their SPR response and silver too susceptible to oxidation. This leaves gold as the most practical metal.
Gold is very resistant to oxidation and other atmospheric contaminants but is compatible with many chemical modification systems. The thickness of the metal layer is of great importance. Above an optimum thickness the dip in reflective light becomes shallow, below the optimum thickness the dip becomes broader (2). The optimal thickness of the gold layer is ± 50 nm.
The light source should be monochromatic and p-polarized (polarized in the plane of the surface) to obtain a sharp dip. All the light which is not p-polarized will not contribute to the SPR and will increase the background intensity of the reflected light (2).
Since the metal film, the incident light and temperature are kept constant in experiments, the SPR signal is directly dependent on the change of the refractive index of the medium on the sensor side of the SPR surface.
Quantitative measurement
The binding of biomolecules on the sensor surface results in the change of the refractive index, which is measured as a change in resonance angle or resonance wavelength.
Fortunately, the change in refractive index on the surface is linear to the number of molecules bound to the sensor surface (11). The linearity holds mainly for protein-protein interactions that have a refractive index increment (RII) of about 0.18-.019 ml/g (12),(13). For other biomolecules, some calibration may be necessary.
Some of the SPR-machines convert the actual measured values (angle or wavelength) into an arbitrary one, which is easy to display and interpret. The BIACORE machine for instance uses the Resonance Unit (RU), which is converted from the actual angle shift in reflected light.
Knowledge of the RII is important for many qualitative and comparative applications of SPR, especially with small molecules. In the process of screening combinatorial libraries, many small molecules are screened at a single concentration. For an accurate affinity ranking and correct stoichiometric measurements, SPR response must be normalized for each compound (12). In addition, accurate RII values are required to estimate film thickness and surface coverage. An additional complication is the nature of the evanescent wave, which will give different absolute values depending on the thickness of the sensor chip surface (14).
The maximal response can be predicted with:
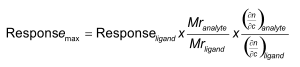
The maximal response (Rmax) assumes that binding is 1:1 and the immobilized ligand is active and fully accessible. The correction for a non 1:1 interaction is:

Where p is the number of binding sites.
Fortunately, the RII is not that important to get meaningful results in simple protein-protein or protein-oligo interactions in which the kinetic constants are determined (14).
Traditional SPR versus multi-parametric SPR
Traditional SPR uses the SPR dip minimum to determine the dip position as function of time. The dip position is presented in a sensorgram. This set-up provides information about the kinetics (ka, kd, KD) and binding level (Rmax). Traditional SPR is widely used.
Multi-parametric SPR determines the SPR dip minimum / position but in addition, records the dip slopes, dip intensity and the surrounding SPR signal (15). This enables multi-parametric SPR to resolve bulk-refractive index differences without a reference channel (inline referencing). This system tolerates up to 5% DMSO and running DMSO calibration is therefore not necessary.
By using a second wavelength layer thickness (Ångström resolution) and RI changes can be detected. Traditional SPR works with thickness up to 150 nm, MP-SPR can work with layers up to several microns thick, allowing work with living cells amongst others.
In addition, MP-SPR is suitable to measure the layer properties (refractive index, thickness, density, extension coefficient, surface coverage, swelling, optical dispersion) of the sample (16),(17),(18).
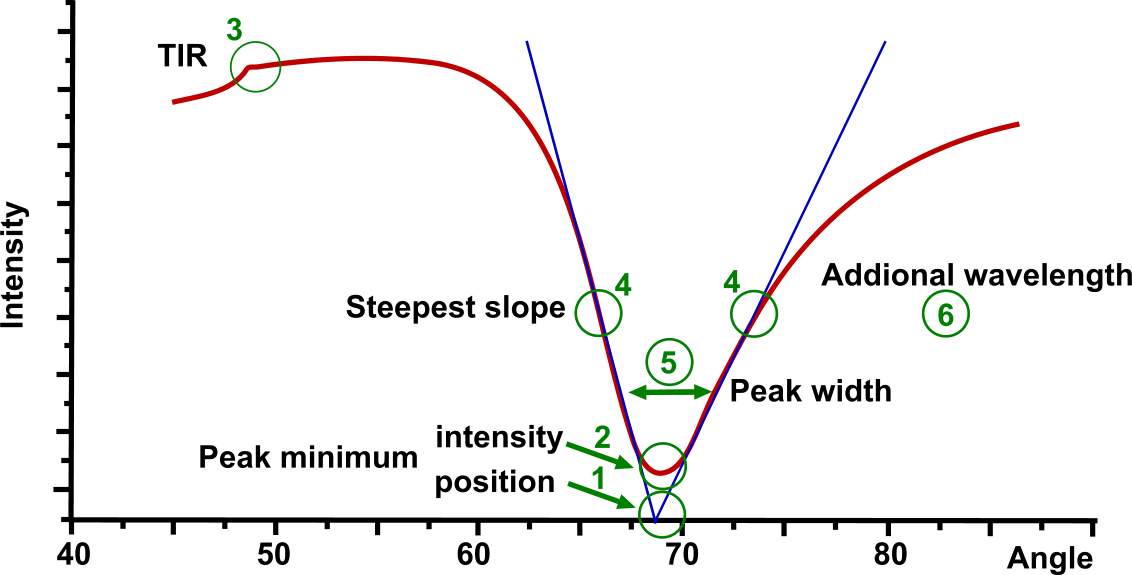
In the Multi-parametric SPR figure several points of the SPR curve are highlighted:
- Peak minimum position is the most used parameter in SPR
- Intensity of SPR peak minimum depends on the sensor material and the media. Unlike others, MP-SPR is able to measure in liquid as well as in gas, on metals, metal oxides and hydrogels.
- Total Internal Reflection (TIR) value is dependent on the bulk properties around the sensor and it is utilized for dn/dc calculation (inline bulk effect).
- Steepest falling and raising slope shows the most sensitive measurement area for the fast fixed angle measurements.
- SPR peak-width at 3 different levels defines the shape of the SPR peak. It contains information on light absorption by the binding molecules and therefore, enables characterization of absorbing coatings and samples.
- Another wavelength pair can be used for all measurements. This resolves layer thickness and RI.
The company BioNavis has a whole range of instruments that use the Multi-parameteric SPR.
References
(1) | Biacore AB BIACORE Technology Handbook. (1994). |
(2) | Nagata, K. and H. Handa Rela-Time Analysis of Biomelecular Interactions. (2000). |
(3) | van der Merwe, P. A. Surface Plasmon Resonance. (2003). |
(4) | Akimoto, T., S. Sasaki, K. Ikebukuro, et al. Effect of incident angle of light on sensitivity and detection limit for layers of antibody with surface plasmon resonance spectroscopy. Biosens.Bioelectron. 15: 355-362; (2000). Goto reference |
(5) | Markey, F. What is SPR anyway? (1999). |
(6) | Quinn, J. G., S. O'Neill, A. Doyle, et al. Development and application of surface plasmon resonance-based biosensors for the detection of cell-ligand interactions. Analytical Biochemistry 281: 135-143; (2000). Goto reference |
(7) | Homola, J. Surface Plasmon Resonance Sensors for Detection of Chemical and Biological Species. Chem.Rev. 108: 462-493; (2008). |
(8) | Roos, H., R. Karlsson, H. Nilshans, et al. Thermodynamic analysis of protein interactions with biosensor technology. J.Mol.Recognit. 11: 204-210; (1998). Goto reference |
(9) | Barr, E. S. Concwerning index of refraction and density. Amer.J.Phys. 23: 623-624; (1955). |
(10) | Eugeniik Surface Plasmon Resonance - a method to analyze interfacial optical properties and to develop biosensors. (2003). |
(11) | Stenberg, E., B. Persson, H. Roos, et al. Quantitative determination of surface concentration of protein with surface plasmon resonance by using radiolabelled proteins. (1991). |
(12) | Davis, T. M. and W. D. Wilson Determination of the Refractive Index Increments of Small Molecules for Correction of Surface Plasmon Resonance Data. Analytical Biochemistry 284: 348-353; (2000). |
(13) | Schuck, P. and A. P. Minton Analysis of mass transport-limited binding kinetics in evanescent wave biosensors. Analytical Biochemistry 240: 262-272; (1996). Goto reference |
(14) | Di Primo, C. and I. Lebars Determination of refractive index increment ratios for protein-nucleic acid complexes by surface plasmon resonance. Analytical Biochemistry 368: 148-155; (2007). |
(15) | Ltd., B. BioNavis SPR Navi. (2012). Goto reference |
(16) | Rupert, D. L. M., G. V. Shelke, G. Emilsson, et al. Dual-Wavelength Surface Plasmon Resonance for Determining the Size and Concentration of Sub-Populations of Extracellular Vesicles. Analytical Chemistry 88: 9980-9988; (2016). Goto reference |
(17) | Kari, O. K., T. Rojalin, S. Salmaso, et al. Multi-parametric surface plasmon resonance platform for studying liposome-serum interactions and protein corona formation. Drug Delivery and Translational Research 7: 228-240; (2017). Goto reference |
(18) | Vuoriluoto, M., H. Orelma, B. Zhu, et al. Control of Protein Affinity of Bioactive Nanocellulose and Passivation Using Engineered Block and Random Copolymers. ACS Applied Materials & Interfaces 8: 5668-5678; (2016). Goto reference |